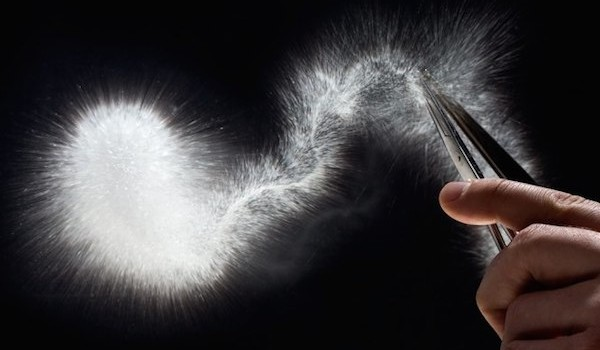
There are many fascinating phenomena in the world of science and engineering, but today I’d like to highlight one in particular: that of Prince Rupert’s Drops, also known as. Prince Rupert’s drops are tear-drop shaped glass beads that generally have very long tails and have extremely unique mechanical characteristics. The play between surface compression and residual internal tensile stresses and the resulting massive amount of stored potential energy makes for quite an interesting show, with the glass fracturing at a crack front propagation rate of somewhere in the neighborhood of 0.9-1.2 miles per second (the equivalent of about 4-6 times the speed of sound, or roughly hypersonic speeds). Before we get our hands dirty with the science behind these drops, a quick word on their history is necessary.
The Drops That the Prince Gave the King
As the story goes, Prince Rupert of the Rhine (a royal duke in 17th century Bavaria, now a part of southeastern Germany) discovered these drops and passed them on to King Charles II of England. King Charles II would eventually go on to task his Royal Society with investigating the scientific reasoning for the drops’ behavior, but before he did, it is rumored that he (as well as Prince Rupert) liked to have a bit of fun with them. Legend has it that the two would use these drops for practical jokes, often placing the bulbous head of the drops in the hands of their subjects and then snapping off the tail of the drop, triggering the chain reaction movement of the fracture, which would result in a (relatively) harmless explosion. While these drops may have been quite fun, the King and Prince were also awed by the scientific mystery associated with the phenomenon and so they began the scientific expedition towards the understanding of these drops, which has now been going on for about 350 years. While the famed father of Elasticity, Robert Hooke, did a lot of early experimentation regarding the behavior of these drops, it was not until the mid 1990s that two engineers from the University of Cambridge and Purdue University used high-speed photography to unravel the secrets of these drops.
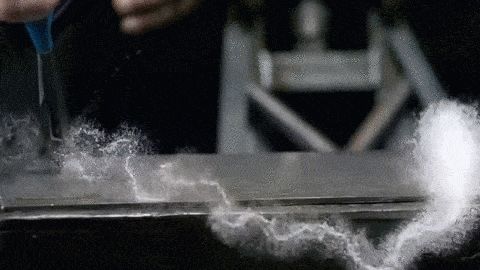
Forming the Drops
What these researchers have uncovered is that the secret of these drops lies in their formation. In order to create these drops, a glass bead is drawn from a furnace and a glob of that bead is allowed to drip from the tool used to remove the molten glass from the furnace into a bucket of water. In doing this, the glass is what we call “water-quenched”, meaning that the glass is cooled quite rapidly in water. This is where the magic starts to happen.
Prior to quenching, the molecules of the molten glass are all spread out relatively evenly and have a fairly high amount of thermal energy associated with them…after all, the glass was just pulled from a furnace. When the glass hits the water, it begins to cool immediately, transferring its heat to the surrounding water and in doing so, solidifying into the teardrop shape seen above. However, this is a slight problem…you see, the outer surface of the glass hits the water first and cools very rapidly, solidifying and essentially insulating the interior of the molten glass drop from the surrounding water. This much should make sense, after all, as most of you are aware, glass has often been used as an insulator, especially in the olden days before better materials and composites were available.
At this point, the exterior has already solidified, and the interior is still molten, yet is cooling to the temperature of the surrounding water, albeit at a much slower rate. Now as this cooling takes place, the glass itself tends to shrink. This occurs because of what is known as thermal contraction (the opposite of thermal expansion), a phenomenon in which most materials will shrink as they cool (and expand as they are heated). When the glass is heated, all of that thermal energy means that the molecules are very excited and move around a lot and generally just take up more space due to their higher activity levels. As the glass cools, much of that thermal energy is lost to the surroundings, reducing the activity level of the molecules and making them move slower and across smaller distances, thus the molecules tend to pull together more, contracting and thus bringing down the total volume that they take up.
To get back on track, remember how we said that the exterior had already cooled and solidified, while the interior had not? Well this means that the exterior has already contracted, but the interior has not. Furthermore, because the exterior cooled so rapidly, the molecules did not have much time to migrate and thus the exterior did not contract all that much because of the lack of time available to do so. The interior, however, is still cooling and contracting all the while and so as the interior cools and contracts, it begins to pull the exterior molecules inwards. This pulling of the drop’s exterior causes the exterior to be forced into a state of compression, as the exterior molecules want to move inwards. As this is happening, the interior molecules are realizing that they are quickly running out of space and their volume is becoming jam-packed and so this results in a state of tension, because they are all pressed up against each other and want to move outwards. By the time the drop has fully cooled and solidified, there is a great deal of residual compression on the exterior and tension on the interior, leading to a very tedious and fragile equilibrium. These residual stresses are quite similar to what is seen in tempered glass (as shown below), but at a higher magnitude.

Breaking the Drops
Now, this external state of residual compression is good news for the drop as glass is very strong in compression and so the combination of these two facts makes the drop very hard to break at the bulbous head, which has a deeper compressive region than that of the relatively thin tail. When you combine these facts with the fact that compression tends to close cracks, whereas tension tends to open them, you can see how it might be difficult to break the bulbous head what with the extensive compressive region. In fact, try as you might, you just simply will not be able to break the bulbous head of the drop…this will remain true even when you hit it hard with a hammer. Having said this, we have to remember that glass is a very brittle material, meaning that it will absorb very little strain (it will deform very little) prior to fracture (as opposed to a very ductile material such as gold which is very malleable and can deform a lot before fracture). Because of this brittleness, when you damage the tail (with its very thin compressive region), you can easily penetrate into the tensile region, which acts to open the crack further.
Now, remember, we said that the internal portion was stressed heavily with a great deal of residual tensile stress. This residual stress is stored in the form of strain energy which is essentially potential energy associated with the straining of the material (caused by the tight packing of the internal molecules, which now want to force themselves outwards to relieve their stress very badly). As soon as the tail is fractured and that first bit of residual internal tensile stress relieves itself, it starts a chain reaction as all of that stored strain energy fights to release itself all at once and the fracture spreads down the line towards the bulbous head of the drop, from the initial fracture at the tail. The rate at which this happens is termed the crack propagation rate and is determined by measuring the speed at which the failure front (or the position of the crack) travels along the drop. Because of the crazy high amount of strain energy left over from the quenching of the drop, the failure front accelerates as it moves from the tail to the head (and then decelerates as it reaches the end of the head) to a maximum speed somewhere between the equivalent of Mach 4-6, or 4-6 times the speed of sound at sea level. The failure front is travelling about as fast as most space vehicle reentry bodies when they re-enter the Earth’s atmosphere. All of this from simply snapping the tail off of a glass drop?
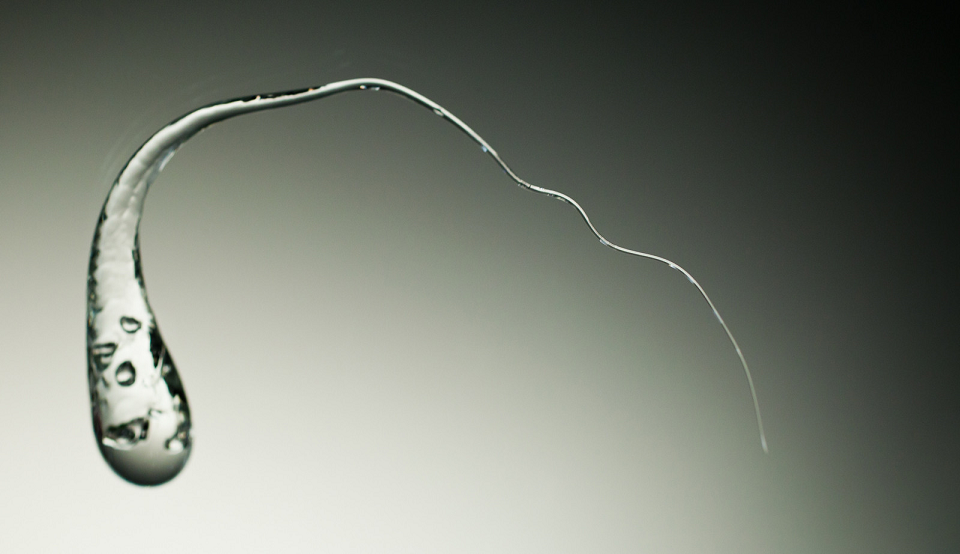
Just another excellent example of how awesome science and engineering can truly be. For some awesome footage of Prince Rupert’s Drop fractures, check out this video from Destin over at Smarter Every Day: click this link for the video.